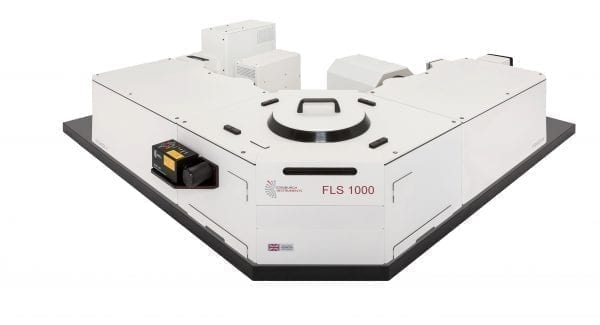
FLS1000 Photoluminescence Spectrometer
- Fluoracle® software package – easily accessible user interface and updated features
- Higher Resolution – 325 mm focal length monochromators provide superb accuracy and excellent stray light rejection with minimum step size
- Faster measurements – increased scan speeds and leading performance
- Increased automation – ease of use for even the most complex configurations
- NEW MicroPL Upgrade allows spectral and time-resolved photoluminescence measurements of samples in the microscopic scale
- Live video demonstration – contact us to arrange an online demonstration. We can accept your samples and talk you through the process as we run test measurements.
- NEW MicroPL Upgrade allows spectral and time-resolved photoluminescence measurements of samples in the microscopic scale
- Live video demonstration – contact us to arrange an online demonstration. We can accept your samples and talk you through the process as we run test measurements.
Product Description
The FLS1000 Spectrometer sets the standard in both steady state and time-resolved photoluminescence spectroscopy for both fundamental research and routine laboratory applications.
The system is a modular fluorescence and phosphorescence spectrometer for measuring spectra from the ultraviolet to the mid-infrared spectral range (up to 5,500 nm), and lifetimes spanning from picoseconds to seconds. All of this can be achieved through various upgrade routes, either at the time of order or in the future.
Whether you are studying photophysics, photochemistry, biophysics, biochemistry, material or life sciences, the FLS1000 will enable you to reliably and accurately measure luminescence spectra and kinetics using state-of-the-art sources, detectors, acquisition techniques, quality optics and precision mechanics. The large sample chamber will house practically any type of sample accessory.
High sensitivity is a prerequisite for measurements of low sample concentrations, small sample volumes or low sample quantum yields. The instrument’s guaranteed sensitivity of >35,000:1 for the standard water Raman measurement using the SQRT method is unmatched in the industry.
The FLS1000 has a USB interface and all modes of operation are controlled by ONE data acquisition module and ONE all-inclusive Fluoracle software package for data acquisition and analysis. Light source, detector, grating, slits, polarisers are all computer-controlled for accurate and precise measurements.
Excitation Sources
The FLS1000 comes standard with a 450 W ozone free xenon arc lamp that covers a range of 230 nm to >1000 nm for steady state measurements. A variety of other sources can be integrated including microsecond flashlamps, nanosecond flashlamp, pulsed diode lasers (EPL Series, HPL Series, VPL Series), pulsed light emitting diodes (EPLED Series, VPLED Series), supercontinuum lasers, Ti:sapphire lasers, Q-switched solid state lasers and OPOs, dye lasers, and infrared CW and pulsed lasers for upconversion measurements.
Monochromators
Single and double grating Czerny-Turner monochromators are available in the FLS1000 with 325 mm (or 2 x 325 mm) focal length, high optical throughput, excellent stray light rejection and low temporal dispersion. The monochromators feature ‚plug-and-play‘ triple grating turrets with up to three gratings on each turret and computer-controlled slits.
Detectors
A full range of detector options are available to enhance the range of spectral coverage and/or to reduce the instrumental response width for lifetime measurements. The instrument comes standard with a PMT-900 detector in a cooled housing which covers a range from 185 nm – 900 nm. In TCSPC mode the instrumental response width is approximately 600 ps. Optional detectors include: high speed PMTs in a cooled housing with instrument response <200 ps, MCP-PMT in cooled housings with a response <25 ps, NIR-PMTs to cover spectral ranges out to 1700 nm with photon counting sensitivity and speed, InGaAs detectors with spectral coverage up to ~1.65 μm, 2.05 um and 2.55 μm, InAs and InSb detectors to cover up to 5.50 μm.
Sample Holders
At the heart of the FLS1000 is an exceptionally large sample chamber that allows access to the sample from all sides, top and bottom. This ensures compatibility and simplifies access to a variety of sample holders.
Photoluminescence Spectroscopy Research
We have a variety of research articles on photoluminescence spectroscopy which you can view on our website.
Enquiries FLS1000 Photoluminescence Spectrometer
For further information, or to find out how the FLS1000 can help you with your photoluminescence spectroscopy work, simply contact a member of our sales team at sales@edinst.com.
Edinburgh Instruments: Photoluminescence spectroscopy solutions for photophysics, photochemistry, material sciences and life sciences.
Technical Specifications
Specifications | Spectral | Phosphorescence Lifetime | Fluorescence Lifetime |
---|---|---|---|
Mode of Operation | Single Photon Counting | Time-Resolved Single Photon Counting (Multi Channel Scaling – MCS) | Time-Correlated Single Photon Counting (TCSPC) |
Lifetime range | Milliseconds to hours | 10 ns – 50 s * | 5 ps – 10 µs * |
Sensitivity | >35,000:1 ** | n/a | n/a |
Excitation Sources | |||
Type | 450 W ozone-free xenon arc lamp | Microsecond flashlamp | Picosecond pulsed diode lasers (EPLs, HPLs) and pulsed LEDs (EPLEDs) |
Spectral Range | 230 nm- > 1000 nm | 200 nm – >1000 nm | Discrete wavelengths between 250 nm – 980 nm |
Pulse width | n/a | 1 µs – 2 µs | from 60 ps |
Options | Ozone generating lamp with spectral range 200 nm – >1000 nm |
Low to medium repetition rate pulsed lasers. Variable pulse width VPL and VPLEDs | Nanosecond flashlamp 200 nm – >400 nm pulse width <1 ns |
* source and detector dependent ** standard water Raman measurement conditions: Excitation wavelength = 350 nm, Excitation and emission bandwidths = 5 nm, step size = 1 nm, integration time = 1 s, emission wavelength = 397 nm, noise measured at 450 nm and calculation based on the SQRT method |
Monochromator | Specifications |
---|---|
Type | Czerny-Turner with ‚plug and play‘ triple grating turret |
Focal Length | 325 mm (double monochromators: 2 x 325 mm) |
Stray Light Rejection | 1:10-5 (single), 1:10-10 (double) |
Gratings | Mounted to triple grating turret |
Accuracy | +/- 0.2 nm * |
Minimum Step Size | 0.01 nm * |
Option | Spectrographs available for operations of CCDs and diode array detectors |
* grating dependent |
Detectors | PMT-900 | PMT-1010 | PMT- 1400/1700 | HS-PMT | MCP-PMT | HS-HPD |
---|---|---|---|---|---|---|
Spectral Range | <200 nm - 870 nm | <200 nm - 1010 nm | 500 nm – 1700 nm | <230 nm - 850 nm | <200 nm - 850 nm | 220 nm – 870 nm |
Dark Count Rate | <50 cps (-20 °C) | <200 cps (-20 °C) | <20 kcps or <200 kps | <150 cps (0 °C) | <10 cps (-20 °C) | <200 cps (10 °C) |
Reponse Width | 600 ps | 800 ps | 400 ps or 800 ps | 180 ps | <25 ps | 20 ps |
Options | A wide variety of other photomultipliers and analogue detectors are available up to 5,500 nm |
Software
Fluoracle spectrometer operating software is at the heart of all our fluorescence spectrometers and is a fully comprehensive, user-friendly data analysis software package. Irrespective of system configurations, this software provides the user with complete control of the instrument and of most third-party accessories.
Fluoracle is Windows compatible and is based on a data centred design that enables the user to focus on their measurement. This guarantees ease-of-use in the operation of a modular and potentially complex spectrometer.
Measurement set-up and data acquisition is made through an intuitive menu system. Key spectroscopic parameters are easily accessed through functional groupings, while common measurement routines can be saved as method files to allow previous experiments to be easily repeated. Tabbed dialogue boxes and particular scan parameters are always visible during set-up. The current status of the instrument is also continuously displayed.
A unique feature of the Fluoracle is that all modes of data acquisition, including spectral scanning and lifetime acquisition in both MCS and TCSPC modes, are controlled from within one software package. Modern light sources, detectors, complex sample holders (plate reader, XY sample stages, titrator) and cooler options (thermostated sample holders and cryostats) are supported and fully software controlled. Fluorescence Lifetime Imaging Microscopy (FLIM) acquisition and analysis are included in Fluoracle with a MicroPL upgrade.
Fluoracle offers the „FAST“ add on for the advanced analysis of fluorescence and phosphorescence decay kinetics.
Measurement Examples (Steady State)
Excitation and Emission Scans
Excitation and emission spectra are standard measurements in fluorescence spectroscopy. The figure demonstrates a measurement of a well documented standard test solution of anthracene in degassed cyclohexane.
Sample: Anthracene in cyclohexane (10-5M). Measurement conditions: λex = 358 nm for emission scan, λem = 400 nm for corrected excitation scan, Δλex = Δλem = 0.4 nm, step size = 1 nm, integration time = 1 s.
Synchronous Scans
In synchronous scans, both excitation and emission monochromators are scanned synchronously with a pre-set offset. The figure demonstrates a sample of five different aromatic hydrocarbons dissolved in cyclohexane, measured with a conventional emission scan (red) and a synchronous scan with zero offset (green). The five hydrocarbons are resolved by the synchronous scan.
Sample: Five aromatic hydrocarbons dissolved in cyclohexane. Measurement conditions: λex = 280 nm for emission scan, Δλex = Δλem = 0.5 nm, step size = 0.5 nm, integration time = 1 s, offset = 0 nm.
Kinetic Scans
Kinetic scans reveal temporal changes of the sample fluorescence at fixed excitation and emission wavelengths. Luminescence emission in the milliseconds to seconds range, such as long phosphorescence, chemical reactions or chemical migration in cells, can be studied. As an example, using the FLS1000 in T-geometry for dual wavelength detection, simultaneous measurements of the Ca2+ active fluorophore Indo-1 can be made with both emission arms set to different wavelengths.
Sample: Human platelets cells loaded with Indo-1 in 1 mM Ca2+. Measurement conditions: λex = 340 nm, λem1 = 485 nm, λem2 = 410 nm, Δλex = Δλem = 1 nm, integration time = 0.5 s.
Excitation – Emission Maps (EEM)
The variety of measurement, display and analysis options allows easy and fast investigation of unknown luminescent samples or samples which contain different fluorophores. One method is to measure a series of emission scans within a selected range of excitation. The result is then demonstrated either in a 3D plot or in a contour plot.
Sample: Three organic dyes in solution: naphtalene, anthracene perylene. Measurement conditions: Xe1, PMT-900, 280 nm ≤ λex ≤ 460 nm, 310 nm ≤ λem ≤ 620 nm, Δλex = Δλem = 2 nm, integration time = 0.5 s, repeats per scan = 1.
Batch Measurements (Batch Mode)
Combinations of excitation, emission, synchronous scans, excitation-emission or synchronous maps can be run in Batch Measurements. This means that several scans can be set for a sample and measured automatically without the presence of the user. The scans can be set to repeat in loops as many times as required, with a fixed pre-set delay between each scan. The batch measurements (protocols) can be saved and loaded for future use.
Temperature Maps
The Fluoracle software can communicate with liquid nitrogen and liquid helium cryostats (along with TE controlled sample holders). Temperature maps can be made by acquiring a series of emission, excitation or synchronous scans for a predefined temperature range. The individual measurements are automatically started when the target temperatures are reached.
Sample: CuInSe2 (a material used for photovoltaic cells). Measurement conditions: Fluoracle-controlled Cryostat, Xe2, PMT-1700, λex = 694 nm, Δλex = 10 nm, Δλem = 5 nm, step size = 1 nm, integration time = 0.2 s. Temperature range: 6 K – 106 K, step 20 K.
Absolute Quantum Yield Measurements
The absolute method for fluorescence quantum yield measurements is becoming more widely used than the relative method, as it does not require a quantum yield standard. This is readily applicable to liquids, films and powders and can be extended into the near infrared spectral range.
The picture shows the independence of the fluorescence quantum yield from the wavelength of excitation for a standard organic dye. The graph shows the area of absorption for eight different excitation wavelengths on the left, while on the right it shows the corresponding emission spectra, scaled by a factor of 5. The inset shows the calculated quantum yields.
Sample: Quinine bisulphate in perchloric acid. Measurement conditions: integrating sphere, Δλex = 5.0 nm, Δλem = 0.5 nm, integration time = 0.3 s.
Singlet Oxygen Emission
The emission of singlet oxygen is known to be very weak and, historically, powerful laser excitation has been used to monitor this. However, both excitation and emission spectra of singlet oxygen can be measured using the FLS1000 with a broadband xenon lamp. The figure demonstrates a measurement of singlet oxygen luminescence generated from erythrosine B in ethanol detected by NIR-PMT (green), and InGaAs (blue) detectors.
Sample: Singlet oxygen generated from erythrosine B in ethanol
Photoluminescence of Lathanides
The electronic configuration of lanthanides enables a wide variety of Stokes and anti-Stokes transitions from the ultraviolet to the mid-infrared. This makes them versatile materials that find widespread use in lasers, solar cells, bio-photonics and sensors. Their intra-4f transitions shielded by the external sub-shell are very sharp and narrow requiring high resolution instruments, as can be seen in the graph below for an erbium-ytterbium doped fluoride. Especially for non-linear processes such as upconversion, powerful lasers are fully integrated with the FLS1000.
Sample: YTa7O19: Er3+-Yb3+ powder phosphor
Other steady state measurement examples: Steady state fluorescence anisotropy, contour plots, water quality assessments, excimer equilibrium, reflection, absorption and quantum yield measurements of phosphor powders, chromaticity and much more.
Measurement Examples (Time-Resolved – TCSPC)
Single and Multiple Exponential Decays
Fluoracle provides analysis tools for standard decay tail fitting and numerical reconvolution. With numerical reconvolution, short lifetime components can be extracted from the raw decay data which would otherwise be distorted or masked by the instrumental profile.
The analysis routine provided is based on the Marquardt-Levenberg algorithm. Up to four exponential decay components can be fitted, with shift and offset fitting as standard. The algorithm is robust, delivers results in a blink of an eye, and is presented in a user-friendly interface.
Additional fit quality parameters are available for quality assessment, such as autocorrelation functions, the Durbin-Watson parameter and standard deviations.
The example shows two measurement results of the same homogeneous solution, taken at two different emission wavelengths. The decay at the shorter wavelength is clearly a single exponential, the decay at the longer wavelength is best characterised by three exponential components.
Sample: Hematoporphyrin IX in phosphate buffer (pH 7.2)
Measurement conditions: EPL 405, MCP-PMT, λex = 398 nm, Δλem = 1.0 nm, rep rate = 1 MHz, λem = 620 nm (left and right graph)
Data analysis: Multi-exponential reconvolution, confidence intervals verified by support plane analysis (FAST). τ1 = 15.02 ± 0.03 ns (left). τ2 = 14.80 ± 0.20 ns, τ2 = 4.62 ± 0.55 ns, τ3 = 0.81 ± 0.20 ns (right).
Time-Resolved Fluorescence Anisotropy
By exciting the sample with vertically polarised light and recording the emission in both the vertical and horizontal plane, one can calculate the fluorescence anisotropy of a homogeneous sample. The fluorescence anisotropy reveals the average rotational diffusion time of the molecules.
The measurement example shows that rotational diffusion in the picosecond time scale can be accurately measured. Most samples show rotational diffusion. To avoid this effect when precise fluorescence lifetime measurements are required, the emission polariser must be set to magic angle conditions, 54.7º (and vertically polarised excitation used).
Sample: POPOP in cyclohexane (left plot: IRF-black, decays with parallel-blue and crossed polariser-red), fluorescence anisotropy (right plot: raw data-green and fit-red). Measurement conditions: EPL 375, MCP-PMT, λex = 375 nm, Δλex = 2.0 nm, λem = 390 nm, Δλem = 2.0 nm.
Data analysis: Full anisotropy reconvolution (FAST) with ellipsoidal rotor model. The rotation diffusion times are 110 ps, 150 ps and 620 ps respectively. A spherical rotor model results in a fit with significantly increased chi-square. POPOP is a rod like molecule.
Other TCSPC measurement examples: Time-resolved emission spectroscopy (TRES), monomer-excimer kinetics, solvent relaxation dynamics and much more.
Measurement Examples (Time-Resolved – MCS)
Time-Resolved Measurements of Lanthanides
The photoluminescence emission lifetime of lanthanides extends over a large time range from nanoseconds to seconds where the method of choice for time-resolved measurements is the MCS technique. Due to the high dynamic range and the accuracy resulting from counting statistics, complex decay analysis can be performed.
The pictures show time resolved measurements from a lanthanide doped glass sample at two different emission wavelengths. At the shorter wavelength the decay is best fitted with three exponential terms, while at the longer emission wavelength the initial rise is followed by a millisecond decay.
Sample: Rare-earthed doped glass
Measurement conditions: μF2, λex = 370 nm, Δλex = Δλem = 2.5 nm, rep rate 100 Hz, step size = 10 nm, spectra produced for every 50 μs (Top Left). μF2, λex = 370 nm, λem = 430 nm, Δλex = Δλem = 2.5 nm, rep rate 100 Hz, step size = 10 nm, measurement time = 2 min (Top Right). μF2, λex = 370 nm, λem = 612 nm, Δλex = Δλem = 1.7 nm, rep rate 20 Hz, measurement time = 8 min (Bottom Left).
Data Analysis: Multi-exponential reconvoltution. Good fit results were achieved with four exponential decay model (Top Right) and model comprising two exponential rise and one decay function (Bottom Left).
Other MCS measurement examples: Time-resolved singlet oxygen measurements, time-resolved FRET measurements and much more.
Upgrades
Configuration Upgrades
Excitation Sources
Monochromator Options
Detector Options
Fluorescence Lifetime Upgrades
Phosphorescence Lifetime Upgrades
Sample Holder Options
Temperature Control Options
Polarisation / Anisotropy
Integrating Sphere
Software Upgrade
MicroPL Upgrade
X-Ray Excited Luminescence
Vacuum Ultraviolet Photoluminescence
Other Upgrades
Application Notes
FLS1000 Photoluminescence Spectrometer
- Photoluminescence Spectroscopy of Carbon Dots
- Energy Transfer in a Liquid Scintillator Investigated using Time-Resolved X-ray Excited Luminescence Spectroscopy
- Time-resolved Spectroscopy of Phosphorescent Oxygen Sensors in a Relevant in vitro Environment for Biomedical Applications
- Measuring Picosecond Fluorescence Lifetimes Using the FLS1000 Equipped with a Hybrid Photodetector
- Fluorescence, Delayed Fluorescence and Phosphorescence Spectra of a TADF Emitter Measured using the FLS1000 with a VPL laser and Gated PMT Detector
You can find all of our Application Notes here.